As the global climate crisis escalates and the transition to a sustainable energy economy crystallizes, the capability for long-term grid-scale storage and conversion of carbon-free energy remains an unmet challenge. Given the intermittent nature of sustainable energy sources, a global phase-out of greenhouse gas-intensive energy sources will require efficient storage and conversion of sustainable energy. In particular, interest is growing in the interconversion of dinitrogen (N2) and ammonia (NH3), given the high energy density of NH3 (~6.25 kWh kg-1).
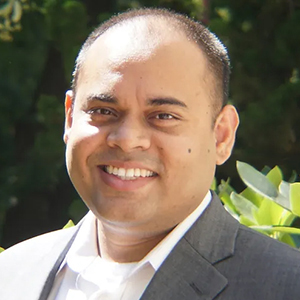
Apart from being a critical fertilizer component, NH3 is also an ideal hydrogen carrier (17.7 wt%, 40% higher than methanol), and can be readily transported and stored using existing infrastructures and then directly utilized for energy applications such as a direct ammonia fuel cell (DAFC). However, today most NH3 is synthesized via the century-old Haber-Bosch process, which operates at extreme temperature (723-823K) and pressure (250-350 atm). It is estimated that NH3 synthesis consumes about 1% of the world’s electricity and a significant fraction of the world’s natural gas for hydrogen production via steam reforming of methane.
Electrochemical synthesis of NH3 from N2 at near-ambient conditions would allow a drastically reduced carbon footprint while simultaneously enabling carbon-free energy storage. The electrochemical N2 reduction reaction (N2RR) is unfortunately hindered by competition with the hydrogen evolution reaction (HER); as a result, despite years of efforts and significant capability and knowledge advances, state-of-the-art N2RR catalysts yield NH3 on the order of μA cm-2, orders of magnitude lower than the DOE REFUEL target of 300 mA cm-2. Furthermore, energy retrieval via the NH3 oxidation reaction (AOR) is kinetically sluggish and requires precious metal catalysts (e.g. Pt, Ir), leading to prohibitively high cost and insufficient performance compared to DOE Targets (>600 mAcm-2 at 0.6 V; efficiency > 60%).
This talk will address the critical need to identify possible alternative routes towards interconversion of N2 and NH3. In the absence of such a capability, a transition to a sustainable energy portfolio will face serious challenges.
Dr. Meenesh R. Singh is an assistant professor in the Department of Chemical Engineering and the director of Materials and Systems Engineering Lab (MaSEL) at the University of Illinois Chicago (UIC), where his research group is developing state-of-the-art computational and experimental tools to solve grand challenges of the 21st century: i) develop carbon sequestration methods, ii) manage nitrogen cycle, iii) provide access to clean water and iv) engineer better medicines.
Dr. Singh obtained his B. E. degree in chemical engineering from Sardar Patel University in 2005, M. Tech. Degree in chemical engineering from Indian Institute of Technology Bombay in 2008, and Ph. D. in chemical engineering from Purdue University in 2013. His research resulted in 7 patents (3 licensed), > 57 publications, 2 book chapters, 5 open-source software, >100 presentations in international conferences, and 28 invited talks. His research lab has been awarded around $2.5 MM in external grant funding with total project funding of $7 MM and has strong/continued collaboration with 7+ multinational companies.
He has mentored 5 Ph.D. students who have recently joined MIT, DTU, UIC, LLNL, and AbbVie, and currently advising 5 Ph.D. students, 3 postdocs, and several undergraduate students. He is a faculty advisor for AIChE Chem-E-Car, editorial board member for 5 scientific journals, including Chemical Engineering Research & Design (Elsevier) and Sustainable Chemistry (MDPI). He is also the CTO of a company, eN-RAMPS, that focuses on the scale-up and commercialization of technologies developed in his lab at UIC.